As discussed previously, polar covalent bonds connect two atoms with differing electronegativities. This results in one atom acquiring a partial positive charge (δ+) and the other atom with a partial negative charge (δ–). The more electronegative atom pulls the electrons towards itself, creating this charge separation. This separation of charge gives rise to a bond dipole moment. The Greek letter mu (µ) represents the magnitude of a bond dipole moment. The formula shown here gives the magnitude of the partial charges, Q. The electronegativity difference determines these partial charges and r represents the distance between them:
μ = Qr
This bond moment represents a vector, a quantity having both direction and magnitude (see figure below). The arrows in dipole vectors point along the bond from the less electronegative atom toward the more electronegative atom. A small plus sign marks the less electronegative end to indicate the partially positive end of the bond. The length of the arrow is proportional to the magnitude of the electronegativity difference between the two atoms.
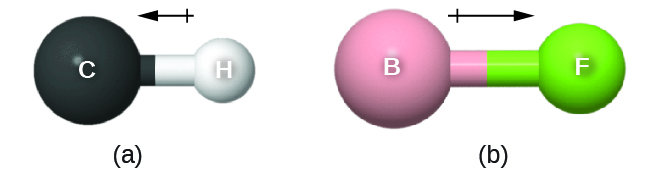
A polar molecule has a separation of charge within its molecular structure. To determine if a molecule is polar we have to look at all the bond dipoles present within the molecule. If no bond dipoles exist, the molecule is nonpolar. If we have bond dipoles present in the molecule we have to look at the addition of these and their orientation in 3D space.
For diatomic molecules, there is only one bond, so its bond dipole moment determines the molecular polarity. Homonuclear diatomic molecules such as Br2 and N2 have no difference in electronegativity, so their dipole moment is zero. For heteronuclear molecules such as CO, there is a small dipole moment. For HF, there is a larger dipole moment because there is a larger difference in electronegativity.
When a molecule contains multiple bonds, the geometry must be considered. If the arrangement of bonds in a molecule causes their bond moments to cancel (resulting in a vector sum of zero), the molecule becomes nonpolar. This is the situation in CO2 ([link]). Each of the bonds is polar, but the molecule as a whole is nonpolar. From the Lewis structure, and using VSEPR theory, we determine that the CO2 molecule is linear with polar C=O bonds on opposite sides of the carbon atom. The bond moments cancel because they point in opposite directions.
In the case of the water molecule ([link]), the Lewis structure again shows that there are two bonds to a central atom, and the electronegativity difference again shows that each of these bonds has a nonzero bond moment. In this case, however, the molecular structure bends because of the lone pairs on O, and the two bond moments do not cancel. Therefore, water does have a net dipole moment and is a polar molecule (dipole).
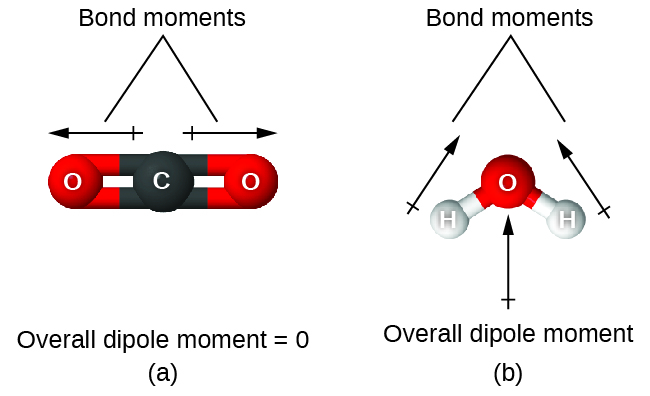
Chloromethane, CH3Cl, is a tetrahedral molecule with three slightly polar C-H bonds and a more polar C-Cl bond. The relative electronegativities of the bonded atoms is H < C < Cl, and so the bond moments all point toward the Cl end of the molecule and sum to yield a considerable dipole moment (the molecules are relatively polar).
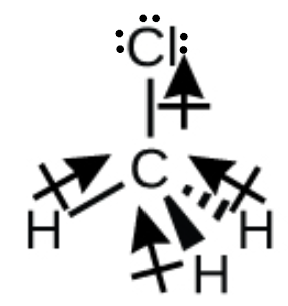
In high symmetry like BF3 (trigonal planar), CH4 (tetrahedral), PF5 (trigonal bipymidal), and SF6 (octahedral), all the bonds have identical polarity (same bond moment). Their geometries are oriented to yield nonpolar molecules, resulting in a dipole moment of zero. Molecules of less geometric symmetry, however, may be polar even when all bond moments are identical. For these molecules, the directions of the equal bond moments are such that they sum to give a nonzero dipole moment and a polar molecule. Examples of such molecules include hydrogen sulfide, H2S (nonlinear), and ammonia, NH3 (trigonal pyramidal).

To summarize, to be polar, a molecule must:
- Contain at least one polar covalent bond.
- Have a molecular structure such that the sum of the vectors of each bond dipole moment does not cancel.
Properties of Polar Molecules
Polar molecules tend to align when placed in an electric field with the positive end of the molecule oriented toward the negative plate and the negative end toward the positive plate ([link]). An electrically charged object can attract polar molecules, but it does not attract nonpolar molecules. Also, polar solvents are better at dissolving polar substances, and nonpolar solvents are better at dissolving nonpolar substances.
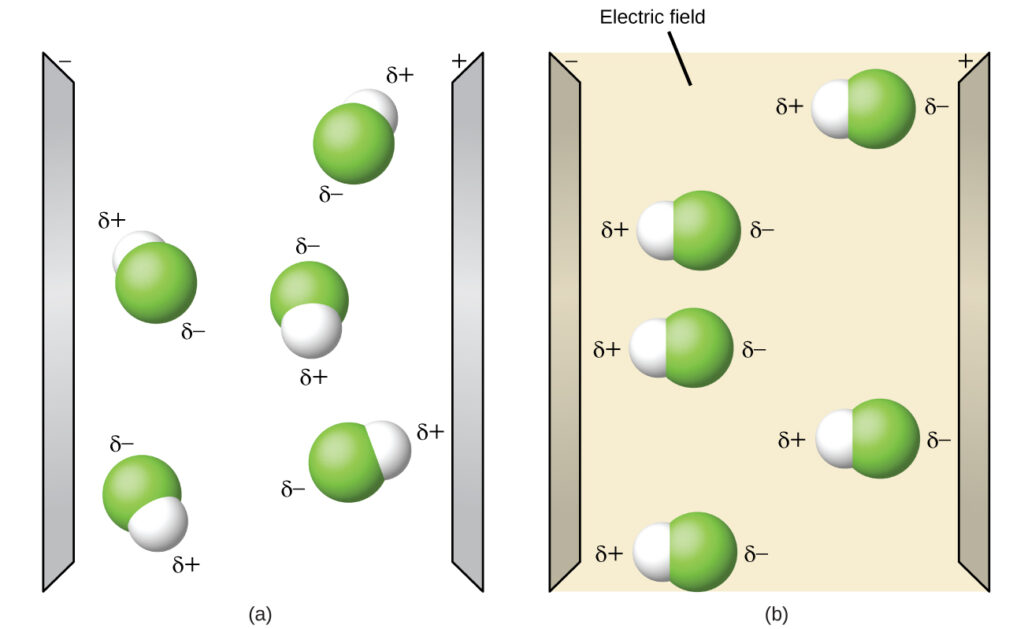
The molecule polarity simulation provides many ways to explore dipole moments of bonds and molecules.
Polarity Simulations
In the simulation, select the “Three Atoms” tab at the top. This should display a molecule ABC with three electronegativity adjustors. You can display or hide the bond moments, molecular dipoles, and partial charges at the right. Turning on the Electric Field will show whether the molecule moves when exposed to a field, similar to the figure above.
Use the electronegativity controls to determine how the molecular dipole will look for the starting bent molecule if:
(a) A and C are very electronegative and B is in the middle of the range.
(b) A is very electronegative, and B and C are not.
Solution
(a) Molecular dipole moment points immediately between A and C.
(b) Molecular dipole moment points along the A–B bond, toward A.
Check Your Learning
Determine the partial charges that will give the largest possible bond dipoles.
Answer:
The largest bond moments will occur with the largest partial charges. The two solutions above represent how unevenly the electrons are shared in the bond. The bond moments will be maximized when the electronegativity difference is greatest. The controls for A and C should be set to one extreme, and B should be set to the opposite extreme. Although the magnitude of the bond moment will not change based on whether B is the most electronegative or the least, the direction of the bond moment will.