Ionic bonding results from the electrostatic attraction between oppositely charged ions, which typically form when metallic atoms transfer electrons to nonmetallic atoms. A different type of bonding results from the mutual attraction of atoms for a “shared” pair of electrons. Such bonds are called covalent bonds. Two atoms form covalent bonds when both have similar tendencies to attract electrons to themselves (i.e., when both atoms have identical or fairly similar ionization energies and electron affinities). For example, two hydrogen atoms bond covalently to form an H2 molecule; each hydrogen atom in the H2 molecule has two electrons stabilizing it, giving each atom the same number of valence electrons as the noble gas He.
Compounds that contain covalent bonds exhibit different physical properties than ionic compounds. Because the attraction between molecules, which are electrically neutral, is weaker than that between electrically charged ions, covalent compounds generally have much lower melting and boiling points than ionic compounds. In fact, many covalent compounds are liquids or gases at room temperature, and, in their solid states, they are typically much softer than ionic solids. Furthermore, whereas ionic compounds are good conductors of electricity when dissolved in water, most covalent compounds are insoluble in water; since they are electrically neutral, they are poor conductors of electricity in any state.
Formation of Covalent Bonds
Nonmetal atoms frequently form covalent bonds with other nonmetal atoms.
For example, the hydrogen molecule, H2, contains a covalent bond between its two hydrogen atoms. [link] illustrates why this bond is formed. Starting on the far right, we have two separate hydrogen atoms with a particular potential energy, indicated by the red line. Along the x-axis is the distance between the two atoms. As the two atoms approach each other (moving left along the x-axis), their valence orbitals (1s) begin to overlap. The single electrons on each hydrogen atom then interact with both atomic nuclei, occupying the space around both atoms. The strong attraction of each shared electron to both nuclei stabilizes the system. As the bond distance decreases, the potential energy also decreases. If the atoms continue to approach each other, the positive charges in the two nuclei begin to repel each other. As a result, the potential energy increases.
The distance at which the atoms achieve the lowest potential energy determines the bond length.
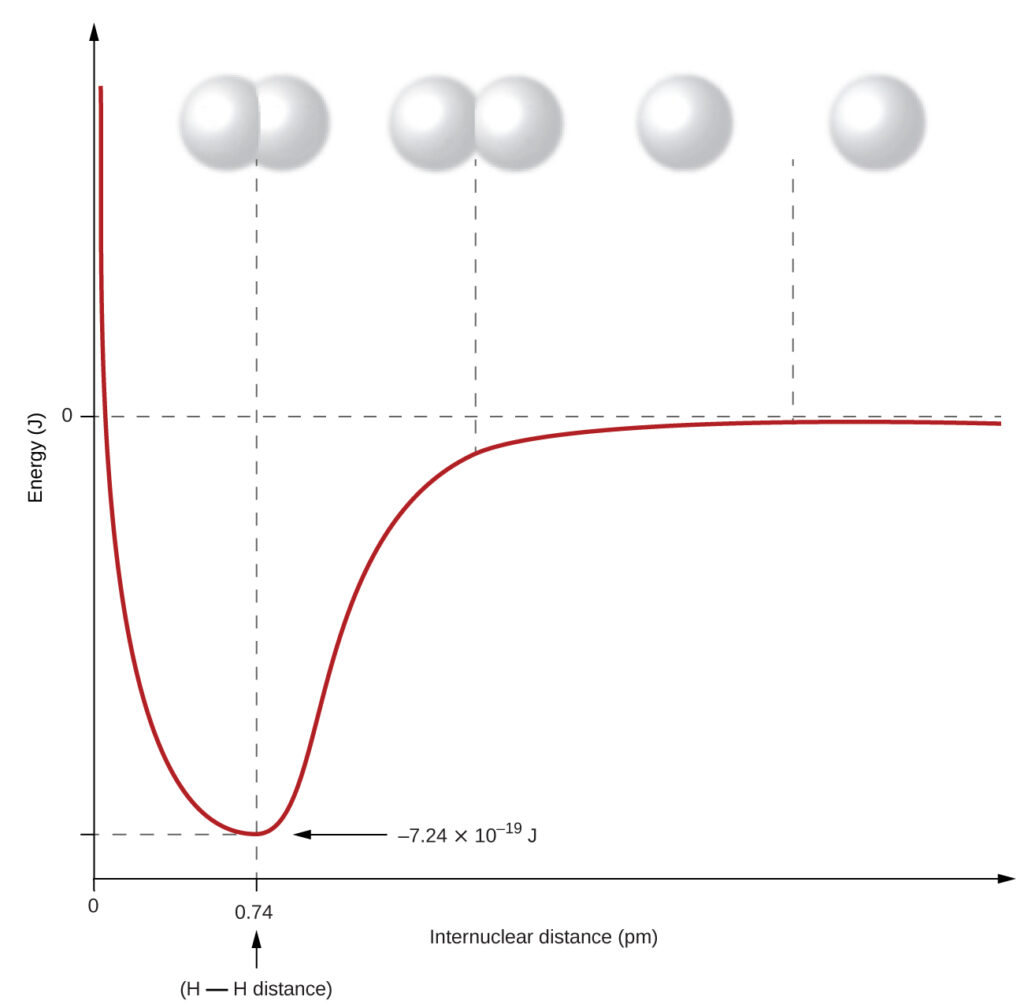
Remember that breaking chemical bons requires adding energy (an endothermic process), whereas forming chemical bonds releases energy (an exothermic process). In the case of H2, the covalent bond is very strong. You must add a large amount of energy, 436 kJ, to break the bonds in one mole of hydrogen molecules and cause the atoms to separate: $$H_2\;(g)⟶2H\;(g)\qquad ΔH=436kJ/mol$$
Conversely, one mole of H2 molecules releases the same amount of energy when it forms from two moles of H atoms: $$2H\;(g)⟶H_2\;(g)\qquad ΔH=−436kJ/mol$$
Pure vs. Polar Covalent Bonds
If identical atoms form a covalent bond, such as in H2, Cl2, and other diatomic molecules, they will share the electrons equally. We refer to this as a pure covalent bond. Electrons shared in pure covalent bonds have an equal probability of being near each nucleus.
In the case of Cl2, each atom starts off with seven valence electrons, and each Cl shares one electron with the other, forming one covalent bond: $$Cl+Cl⟶Cl_2$$
The total number of electrons around each individual atom consists of six nonbonding electrons and two shared (i.e., bonding) electrons. This adds up to a eight total electrons, matching the number of valence electrons in the noble gas argon. Since the bonding atoms are identical, Cl2 also features a pure covalent bond.
When different atoms form a covalent bond, they sare the bonding electrons unevenly. Instead, the bonding electrons are more attracted to one atom than the other. This attraction causes a shift of electron density toward that atom. We call this unequal distribution of electrons a polar covalent bond. A partial positive charge appears on one atom, while the other atom carries a partial negative charge. The atom that attracts the electrons more strongly acquires the partial negative charge and vice versa.
For example, the electrons in the H–Cl bond of a hydrogen chloride molecule spend more time near the chlorine atom. Theyspend less time near the hydrogen atom. Thus, in an HCl molecule, the chlorine atom carries a partial negative charge. Meanwhile, the hydrogen atom has a partial positive charge. [link] shows the distribution of electrons in the H–Cl bond. Note that the shaded area around Cl is much larger than it is around H. Compare this to [link], which shows the even distribution of electrons in the H2 nonpolar bond.
We sometimes designate the positive and negative atoms in a polar covalent bond using a lowercase Greek letter “delta,” δ. A plus sign (δ+) to indicates a partial positive charge, while a minus sign (δ–) to shows a partial negative charge. This symbolism is shown for the H–Cl molecule in [link].
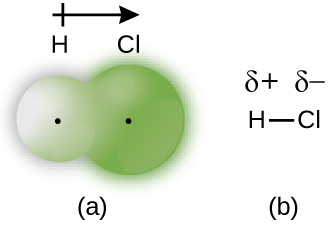