The electron affinity (EA) is the energy change for the process of adding an electron to a gaseous atom to form an anion (negative ion). This process is represented by the equation: $$X\;(g)+e^−⟶X^−(g)$$
This process can be either endothermic or exothermic, depending on the element. Figure 1 shows the EA values for some elements. Many of these elements have negative EA values, indicating that they release energy when the gaseous atom accepts an electron. However, for some elements, adding an electron requires energy, making their EA positive. Just like with ionization energy, forming ions with more charge requires increasingly higher EA values. The second EA involves the energy associated with adding an electron to an anion to form a –2 ion, and so on.
As we might predict, it becomes easier to add an electron across a series of atoms. This is because the effective nuclear charge of the atoms increases. We find, as we go from left to right across a period, EAs tend to become more negative. Exceptions found in group 2 (2A), group 15 (5A), and group 18 (8A) arise from their electronic structures. The noble gases in group 18 (8A) have a completely filled shell. Adding an electron requires placing it in a higher n level, which is more difficult to do. Group 2 (2A) has a filled ns subshell, and so the next electron added goes into the higher energy np. Therefore, the observed EA value is not as the trend would predict. Finally, group 15 (5A) has a half-filled np subshell and the next electron must be paired with an existing np electron.
In all of these cases, the initial relative stability of the electron configuration disrupts the trend in EA.
Electron Affinity of Fluorine vs. Chlorine
We also might expect the atom at the top of each group to have the most negative EA. This expectation is based on their first ionization potentials suggest that these atoms have the largest effective nuclear charges. However, as we move down a group, this pattern changes. In fact, the second element in the group most often has the most negative EA. The small size of the n = 2 shell and the resulting large electron–electron repulsions cause this. For example, chlorine, with an EA value of –348 kJ/mol, has the highest value of any element in the periodic table. The EA of fluorine is –322 kJ/mol.
When we add an electron to a fluorine atom to form a fluoride anion (F–), we add an electron to the n = 2 shell. The nucleus attracts the electron, but other electrons in the small valence shell create significant repulsion. The chlorine atom has the same electron configuration in the valence shell. However, the entering electron is going into the n = 3 shell, which occupies a considerably larger region of space. This larger shell reduces electron-electron repulsions so the entering electron does not experience much repulsion. As a result the chlorine atom accepts an additional electron more readily, resulting in a more negative EA.
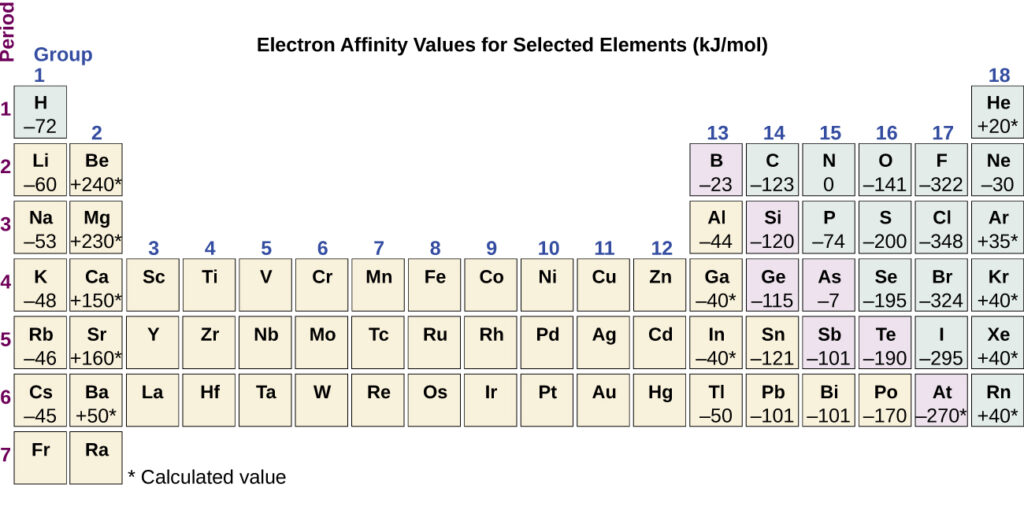
The properties discussed in this section are central to understanding chemical reactivity. These include size of atoms and ions, effective nuclear charge, ionization energies, and electron affinities.
For example, fluorine has an energetically favorable EA and a large energy barrier to ionization (IE). Thus, it is much easier to form fluorine anions than cations.
Metallic properties like conductivity and malleability, ability to form sheets, depend on the ability to easily remove electrons. Thus, metallic character increases as we move down a group and decreases across a period, following atomic size trend. This is because it is easier to remove an electron that is farther from the nucleus.
Key Concepts and Summary
Electron configurations allow us to understand many periodic trends. Covalent radius increases as we move down a group because the n level (orbital size) increases. Covalent radius mostly decreases as we move left to right across a period because the effective nuclear charge experienced by the electrons increases, and the electrons are pulled in tighter to the nucleus. Anionic radii are larger than the parent atom, while cationic radii are smaller, because the number of valence electrons has changed while the nuclear charge has remained constant. Ionization energy (the energy associated with forming a cation) decreases down a group and mostly increases across a period because it is easier to remove an electron from a larger, higher energy orbital. Electron affinity (the energy associated with forming an anion) is more favorable (exothermic) when electrons are placed into lower energy orbitals, closer to the nucleus. Therefore, electron affinity becomes increasingly negative as we move left to right across the periodic table and decreases as we move down a group. For both IE and electron affinity data, there are exceptions to the trends when dealing with completely filled or half-filled subshells.